Introduction
Far-red light has been something of a controversial topic in commercial horticulture over the last five years. Regardless of crop, growers are expressing a strong curiosity on, if not outright desire to add far-red to their lighting strategies. As more and more lighting providers have new far-red offerings in their product portfolio without providing clear justification how such offerings may or may not help growers, I’d like to provide a bit of guidance on the topic, looking through the lenses of horticultural science, plant biochemistry, and marketing.
Over the next few weeks, I’ll be addressing far-red concepts starting with the basics, then extending into more advanced concepts how the Emerson Enhancement Effect works (and if it even matters in commercial horticulture) and how far-red induces complex biochemical signal cascades that result in cell expansion. Toward the end of the series, I’ll be summarizing all the projects Fluence has executed studying far-red applications to date, including work in tomato, cucumber, strawberry, pepper, leafy greens, and cannabis. I’ll be ending the series of articles with a bit of reflection on some of the more questionable opinions and statements that are propagated in the market, hoping that I can better equip growers to separate credible information from misinformation.
Without further preamble, I’m going to begin our far-red deep dive series by addressing the fundamentals of far-red, as well has how far-red is implicated in photosynthesis and yield. Enjoy!
Fundamentals
Far-red refers to wavelengths of light between 700 and 800 nm. This falls just outside the range of visible light for humans and what is traditionally considered “photosynthetically active radiation” (PAR) for plants. In horticulture, more PAR generally correlates with more yield because PAR reliably drives photosynthesis, and more photosynthesis implies more CO2 turned into plant material.
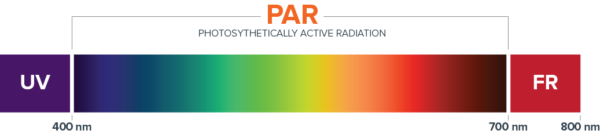
Figure 1: Far-red light spans 700-800 nm, just outside the range of “Photosynthetically Active Radiation”.
Far-red light is foremost used in horticulture to induce morphological responses. Far-red can induce dramatic expansion manifesting as leaf expansion in leafy greens like lettuce and basil, hypocotyl and internode extension in young vegetative plants, and rapid vine stretching in vine crops like tomato, cucumber, and pepper. Far-red can also be used to trigger or suppress flowering in some species, useful in managing ornamental production.
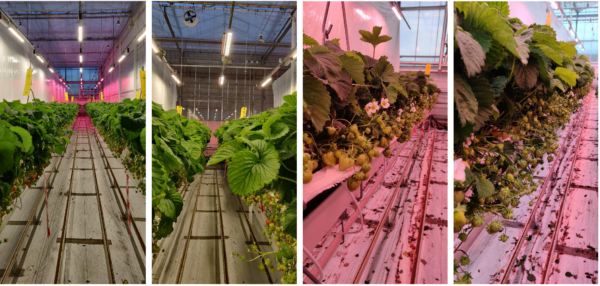
Figure 2: Strawberry plants grown under white or pink light, each with or without some far-red included in the spectrum. From left to right: white; white+FR; pink; pink+FR. The canopy is noticeably expanded with the FR treatments, resulting in larger leaves, better canopy air circulation, and better visibility and access to the fruit.
A secondary, less well defined or understood use of far-red is in improving yields. This is done through indirect means that will be elaborated on later in this series, but generally include supporting photosynthesis, encouraging source-to-sink carbon translocation, and increasing leaf area to intercept more light, thereby capturing more energy for photosynthesis.
Understanding how far-red impacts yield
Regardless of crop, many commercial growers believe that spectra with FR are going to achieve greater yields than those without. Several factors feed this perception:
- The published research is difficult to interpret, poorly communicated to non-scientists, and often has seemingly contradictory findings
- Popular concepts like the so-called “Emerson Enhancement Effect” (EEE) are confusing and generally misunderstood
- Some individuals or businesses in photobiology monetarily benefit from these perceptions so aren’t motivated to say anything to the contrary.
This positive perception of FR-for-yield also seems to be amplified by an echo-chamber effect in our industry:
- Early research on FR that wasn’t executed with commercial considerations in mind had visually compelling, easy to understand results. Bear in mind, far-red’s greatest impact on plant development is morphological, so seeing the differences in plants grown with or without far-red is visually striking, and generates more curiosity about the technology. Thus, these early, commercially irrelevant studies reasonably inspired intrigue amongst commercial growers.
- Commercial interest spurred lighting manufacturers to offer lighting solutions that incorporated FR and leveraged the aforementioned early research to help sell these new products.
- Grower adoption of these products encouraged more productization. With the availability of more FR products, more research is executed (which is starting to be commercially relevant), and commercial interest is driven to (what I believe) is a peak.
- We are now at an inflection point, or perhaps a dividing point, where enough commercially relevant research has been executed to reveal where FR is and is not valuable. Some lighting manufacturers are now being more selective in how/if they produce and promote FR products, while others continue to promote a genetic FR+positive narrative.
The biggest driver of a broadly positive FR-yield perception (and also the biggest hurdle in overcoming this perception) is that in certain conditions, FR photons can be as effective as PAR photons in driving yield while also inducing canopy expansion, creating the appearance of greater yields compared to PAR-only spectra.
There are certain very specific and uncommon conditions where FR photons will be more effective than PAR photons in creating biochemical energy in plants, but FR can’t do it alone, and most of the time using FR will be a more expensive, less reliable lighting strategy for growers than just using PAR light.
History of FR in Horticultural Lighting, and the Challenges of Interpreting FR Research
Studying the effects of FR light on horticultural crops and then interpreting and reporting your observations is considerably more challenging than with PAR light. The first challenge lies in normalizing the amount of light being applied to plants between treatments. For instance, you could choose to normalize photon flux density (PFD) based on the traditional definition of what light is considered photosynthetically active radiation (PAR). Thus, you would term this intensity, “photosynthetic photon flux density” (PPFD) and you wouldn’t include any photons of FR wavelengths that fall outside of PAR. In such a scenario, any FR applied would be extra energy added on top of PPFD. Conversely, you could choose to normalize on the recently coined “ePAR” range of photons that spans 400 nm to 750 nm (Zhen et al., 2019, 2021; Zhen & Bugbee, 2020)
Historically, most photobiological research has normalized flux densities considering only PAR photons. This means that, in any studies where FR was also being applied in a way that would be photosynthetically relevant, it has been ignored as a contributing factor, resulting in some very skewed, misleading findings. Similarly, if you were to compare two horticultural lamps that should have roughly the same total photon output, but one of those lamps emits 10% FR, that lamp would be under-estimated in its ability to drive photosynthesis… though by how much it is under-estimated is hard to generalize.
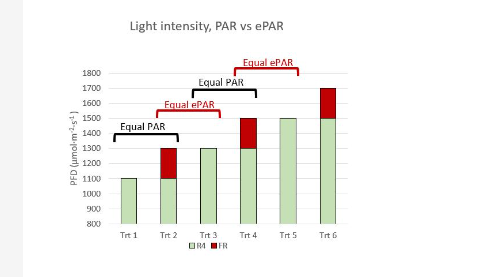
Figure 3: Light intensity treatments normalized on either PAR or ePAR. Ambiguity in how FR photons are quantified amongst the light applied to plants has resulted in confusing, hard to interpret historical research.
PAR vs ePAR
It has recently been proposed that a new range of wavelengths become the standard when discussing the photosynthetic efficacy of horticultural lights. The so-called “ePAR” range spans 400 nm to 750 nm, including wavelengths up to 50 nm longer than the 700 nm cut-off of the PAR range.
In most practical, commercially relevant horticultural lighting situations, ePAR is indeed a more appropriate standard. Note however, ePAR is not a replacement for PAR; there are some small, but critical differences in how these terms are defined.
ePAR accommodates and treats FR photons as equal contributors to overall photosynthesis compared to PAR light, assuming context of FR light being applied in conjunction with PAR light or sunlight. Unlike any of the wavelengths defined in the range of PAR, far-red cannot drive photosynthesis on its own. In many circumstances, however, when applied in combination with PAR light, FR can improve photosynthetic efficiency to the point of achieving comparable levels of photosynthesis as what would have been achieved by adding the same amount of PAR light. There are even circumstances, rare though they may be in commercial horticulture, where FR can actually promote greater photosynthetic rates than what could be achieved with PAR photons. This phenomenon is known as the “Emerson Enhancement Effect” (EEE) (Govindjee, Owens, and Hoch, “A Mass-Spectroscopic Study of the Emerson Enhancement Effect”; Govindjee, “Emerson Enhancement Effect in Chloroplast Reactions”; Zhen and Bugbee, “Far-Red Photons Have Equivalent Efficiency to Traditional Photosynthetic Photons”; Zhen and Iersel, “Far-Red Light Is Needed for Efficient Photochemistry and Photosynthesis.”).
The Emerson Enhancement Effect
For growers that would like to better understand how and when FR may be most effective in driving photosynthesis in their crop (and note: this does not necessarily equal driving yield) it helps to have a clear understanding of the EEE as it pertains to the light reactions of photosynthesis.
The relevant light reactions of photosynthesis can be summed up as follows:
- Input energy (PAR light) excites a protein complex called photosystem II (PSII).
- PSII harvests an electron from water.
- The electron is passed from carrier to carrier via an electron transport chain.
- The electron is passed to photosystem I (PSI)
- And then finally passed to ferredoxin-NADP reductase (FNR) where it is finally consumed in the creation of biochemical energy, NADPH.
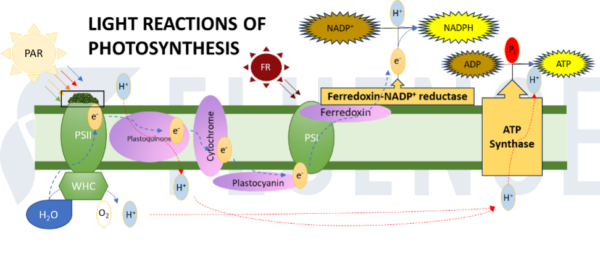
Figure 4: The light reactions of photosynthesis. PAR light is the primary energy input to this process, catalyzing the harvesting of water. Far-red is primarily implicated in providing an “energetic boost” at Photosystem I, allowing more efficient production of NADPH.
If you apply more PAR light, you are pushing more energy into the front of this system. To a point, the rate of photosynthesis will remain proportional to the amount of input energy on the front end. Eventually though, inefficiencies in this system, specifically the rate at which the electron transfers from PSI to FNR in step #5 above begin to bottleneck this process. It is at this breakpoint that FR becomes most valuable in promoting photosynthesis. Far-red light energizes PSI, enabling PSI to more efficiently transfer electrons from PSI to FNR.
To make an analogy, imagine photosynthesis is like pushing water out of a syringe. Adding PAR light is like adding pressure to the plunger of the syringe. The harder you press, the faster water is pushed out, but there is a point of diminishing returns with that strategy. Eventually, it is better to increase the diameter of the needle on the syringe than to keep pushing harder on the plunger. Using far-red light is like increasing the diameter of the needle. By itself, it’s not going to push the water out of the syringe. But deployed appropriately with PAR light, it can make the system more efficient.
So, is ePAR relevant for growers?
Sort of. For most commercial growers, the conditionality of ePAR, and how effective (or not) far-red is in improving photosynthesis is technical hair-splitting.
- In most commercially relevant horticultural light spectra,
- Applied at most commercially relevant light intensities,
- And in most commercially relevant production environments,
It is reasonable for a grower to assume that up to a certain, relatively low photon flux density, FR photons will be equally effective in promoting photosynthesis as PAR photons. What a grower should absolutely not assume is that promoting photosynthesis is the same as promoting yield…
Photosynthesis vs yield
By definition, photosynthesis refers to fixing carbon dioxide from the atmosphere into plant biomass. One might logically extrapolate this to conclude that higher photosynthetic rates would always translate to greater crop yields, but this isn’t always the case, especially with FR. Photosynthates (i.e. the sugars produced in photosynthesis) don’t all end up in the fruiting bodies of crops. In fact, if FR were used to promote photosynthesis in a tomato crop, it is vitally important to consider also the morphological effect that far-red has on that crop. Recall from earlier, far-red sends a strong signal to vine crops to stretch! Thus, it can be the case that many of the photosynthates FR helped produced are consumed in the production of vegetative vine biomass instead of the tomato fruit. The same can be said for cucumbers, where in Fluence’s own research, we’ve observed FR to induce significant leaf expansion at the cost of fruit yield. In both scenarios, yield would have been better served by PAR light, and FR would be better utilized purely for crop steering, inducing morphological changes when required.
There are some cases where ePAR light can be superior to PAR light for yield. Lettuce is a crop where the FR morphological queue to expand its leaves combines nicely with leaf photosynthesis to often generate more harvestable biomass than what would be achieved without FR. Strawberry yields also significantly benefit from FR, though the mechanisms are more complex. FR’s “stretch” queue reduces strawberry canopy density, allowing better airflow, pollination, improves access and visibility to, inflorescences, and leads to greater light interception by the leaves to drive photosynthesis, all leading to greater berry yields.
PAR and ePAR: Conceptually flawed to begin with
It is worth understanding though that even with all these caveats, both PAR and ePAR are conceptually sloppy and can be very misleading. For example, cyan light and red light are both in the range of what is considered photosynthetically active radiation, yet if you were grow a plant under each of these colors at equivalent photon flux densities, they would develop into very different plants. Similarly, growing a plant with a very high fraction of FR compared to a plant grown with a very high fraction of blue of equal flux densities will certainly not be equal plants, even though blue and FR are both ePAR. Understanding total spectral makeup and how it relates to your crop, environment, and production objectives is critically important to understand; one should never make a horticultural lighting decision based only on how much PAR or ePAR a fixture will apply.
Conclusions and Takeaways
And so concludes our first steps into reviewing all things far-red. To summarize:
- Far-red light (700 – 800 nm) is just outside the range of photosynthetically active radiation (400 – 700 nm).
- Far-red is implicated in plant photosynthesis and morphology.
- Historical photobiological research comparing spectral responses is confusing and hard to interpret because the work erroneously did not include far-red as part of the total photon flux applied to the plants.
- Historical FR research is hard to interpret because of how conditional FR’s contribution to photosynthesis is.
- ePAR is not a replacement for PAR but is an important metric to understand.
- The Emerson Enhancement Effect describes scenarios where the light reactions of photosynthesis are saturated with PAR light. In such a scenario, adding more PAR light will not proportionally increase photosynthesis. Adding FR instead can improve photosynthetic rates in these scenarios by improving the flow of electrons through photosystem one, improving total system efficiency. In the vast majority of commercial horticultural productions, growers are not close to photosynthetic satursation, and thus would not reap any enhancing effect from FR; growers seeking to increase photosynthesis should instead simply add more PAR light.
- Increasing photosynthesis doesn’t always translate to increasing yield. For example, if you were to use FR to increase photosynthesis in a cucumber crop, you would also be telling the plant to stretch. Those two things in combination, the cucumber vine will photosynthesize more, and those photosynthates will most likely be consumed in producing more vine or leaf tissue rather than producing more fruit.
- PAR and ePAR are poorly defined concepts that imply equality across photosynthetically relevant photons, when really photosynthetic activity is quite different across different wavelengths. A spectrum decision for horticulture should never be based simply on how much PAR or ePAR a spectrum has, but instead in clearly understanding exactly how a spectrum affects a given crop.
References
- Govindjee, Owens, O. v. H., & Hoch, G. (1963). A mass-spectroscopic study of the Emerson enhancement effect. Biochimica et Biophysica Acta, 75(Supplement C), 281–284. https://doi.org/10.1016/0006-3002(63)90611-5
- Govindjee, R. (1964). Emerson enhancement effect in chloroplast reactions. Plant Physiology, 39(1), 10.
- Zhen, S., & Bugbee, B. (2020). Far-red photons have equivalent efficiency to traditional photosynthetic photons: Implications for redefining photosynthetically active radiation. Plant, Cell & Environment, 43(5), 1259–1272. https://doi.org/10.1111/pce.13730
- Zhen, S., Haidekker, M., & van Iersel, M. W. (2019). Far-red light enhances photochemical efficiency in a wavelength-dependent manner. Physiologia Plantarum, 167(1), 21–33. https://doi.org/10.1111/ppl.12834
- Zhen, S., & Iersel, M. V. van. (2017). Far-red light is needed for efficient photochemistry and photosynthesis. Journal of Plant Physiology. https://doi.org/10.1016/j.jplph.2016.12.004
- Zhen, S., van Iersel, M., & Bugbee, B. (2021). Why Far-Red Photons Should Be Included in the Definition of Photosynthetic Photons and the Measurement of Horticultural Fixture Efficacy. Frontiers in Plant Science, 12. https://doi.org/10.3389/fpls.2021.693445
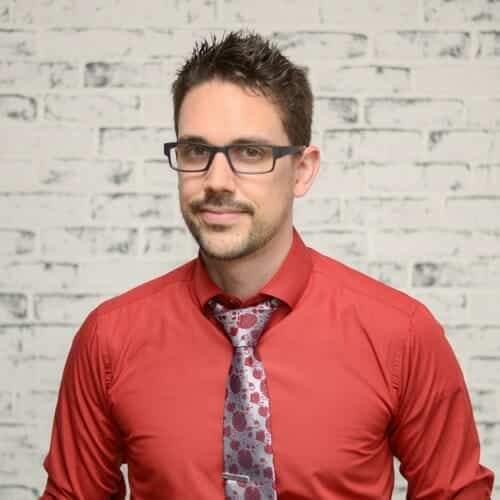
DR. DAVID HAWLEY
Lead Scientist
Dr. David Hawley leads the scientific research initiative at Fluence as the company’s principal scientist. His experience in controlled environment systems, horticultural lighting and cannabis metabolome naturally underpins Fluence’s mission to drive industry-leading lighting research to explore the interaction between light and life.